Sandbox Reserved 702
From Proteopedia
Anthrax edema factor (EF)
| |||||||||
1lvc, resolution 3.60Å () | |||||||||
---|---|---|---|---|---|---|---|---|---|
Ligands: | , , | ||||||||
Activity: | Adenylate cyclase, with EC number 4.6.1.1 | ||||||||
Related: | 1k90 | ||||||||
| |||||||||
| |||||||||
Resources: | FirstGlance, OCA, PDBsum, RCSB | ||||||||
Coordinates: | save as pdb, mmCIF, xml |
Anthrax edema factor (EF) is an enzyme which is part of the Bacillus anthracis anthrax toxin. Here we study 1lvc – EF adenylate cyclase domain + calmodulin + anthraniloyl-deoxy-ATP.
Introduction
The edema factor is a calmodulin-dependent adenylate cyclase. Adenylate cyclase [ATP pyrophosphate-lyase (cyclizing), (ADCY, EC number 4.6.1.1)] increases intracellular cyclic AMP (cAMP) concentrations in eukaryotic cells.
In fact adenylyl cyclase catalyzes the conversion of adenosine triphosphate (ATP) into cAMP and pyrophosphate. The cellular level of cAMP increases, upsetting water homeostasis and causing disruption of signaling pathways.EF is produced in an inactive form. When it is in the cell, EF Adenylyl cyclase activity is induced by complexation with calmodulin, so it is allosterically activated. Its enzymatic activity leads to a dramatic elevation of the cAMP range. Calmodulin is ubiquitous eukaryotic cellular protein and a Ca2+ ion sensor present in host cells. [1]
Cyclic AMP is a second messenger that plays key roles in the signal transduction pathways and thus regulates diverse cellular responses. It binds to three families of signal transducers:
- cAMP-dependent protein kinases
- cyclic nucleotide gated channels
- guanine nucleotide exchange factor for Ras GTPase homologs Rap1 and Rap2. [2] [3]
Anthrax toxin
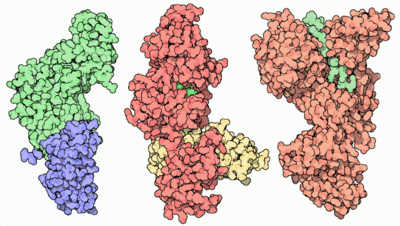
The anthrax toxin is composed of a cell-binding protein (protective antigen) (83kDa), lethal factor (90kDa) and edema factor (89kDa). Edema factor, protective antigen and lethal factor can also be called factor I, II and III respectively. [5] [6]
The complex produced by Bacillus anthracis consists of a virulent mixture of two toxins, with different enzymatic activities. They have a similar N-terminal domain that allows them to bind to a large protein: Protective Antigen.
- Protective antigen (PA)
PA binds mammalian receptors CMG2 (capillary morphogenesis gene 2) and TEM8 (tumor endothelial marker 8). PA promotes EF and LF translocation in the cytosol of infected cells, particularly macrophages, where the two enzymes perform their damage-inducing processes, allowing bacteria to evade the immune system. [7]
- Lethal factor (LF)
LF is a zinc-mediated metalloprotease that cleaves mitogen-activated protein kinase kinases (MEKs). This impairs cell signaling, and results in the induction of apoptosis. [8]
- Edema factor (EF)
EF is a calmodulin-dependent adenylate cyclase that depletes cellular adenosine triphosphate (ATP) while creating 3',5'-cyclic adenosine monophosphate (cAMP), a cellular second messenger. [9] Both toxins can be reconstituted vitro, by combining PA with LF or EF, to form lethal toxin (LT) or edema toxin (ET).
- Edema toxin (ET)
ET is the combination of PA and EF, inducing pathogenic effects. PA is the dominant antigen for immunization, that’s why it is more studied for therapeutic efforts, but LF and EF are also targeted as they are important effectors during anthrax infection.
Associated disease
Bacillus anthracis is the bacterium that is the causative agent of anthrax. The virulence of Bacillus anthracis, Gram-positive bacterium, is mediated through its poly-D-glutamic acid capsule and its toxin. Bacillus anthracis can form very resistant spores that can survive for decades and spread easily. Anthrax is both a disease and bioterrorism threat.
Anthrax is an acute disease, which means that it has a rapid onset and a short course. Most forms of the disease are lethal, and it affects both humans and animals. Anthrax can be contracted in laboratory accidents or by handling infected animals.
Anthrax spores can be produced in vitro and used as a biological weapon to intentionally infect people. As an example, letters containing anthrax spores were mailed to news media offices and U.S. Senators, killing 5 people and infecting 17 others in 2001 in the United States. [10]
Different forms of disease
Anthrax develops when the organism enters in contact with spores of Bacillus anthracis. Symptoms are depending on the contamination mode. The cutaneous contamination results from a contact of spores with an injury. It leads to an ulcer and to the formation of vesicles. In 80 % of the cases the wound heals without complications. However, sometimes an oedema can develop itsel and grow. In that case, anthrax can lead to the death of the patient.
A gastrointestinal contamination can result from the consumption of contaminated meat. This form of anthrax leads to ulcers, nauseas, diarrhoea and blood poisonning. It can also be lethal if it is not rapidly treated.
Finally, anthrax spores can cause a pulmonary infection by inhalation. The symptoms developed are similar to those of influenza and they evolve into breathing difficulties and hypotension. Blood poisonning and meningitis can also occur. Because of the severe symptoms, the pulmonary infection remains highly lethal.[11] The mortality is caused by the combined effects of bacterial toxins (toxaemia) and bacterial growth (becteremia).
Action of the toxin
The secreted proteins can produce two toxic actions. The protective antigen associated with the lethal factor forms the lethal toxin wile associated with the edema factor it forms the edema toxin. The lethal toxin is involved in the bacterial virulence. The edema toxin plays a key role in anthrax pathogenesis by modulating functions necessary for immunity. [12]
Injection of the lethal toxin causes death of rats, whereas the edema toxin causes oedema in the skin of guinea pigs.[13]
Since the edema factor secreted by Bacillus anthracis leads to a massive cAMP formation, it affects intracellular signalling pathways. This factor may also play a key role in anthrax pathogenesis by disrupting the host cell's defence against bacterial infection. The toxin has effects on macrophages, dendritic cells, neutrophils, endothelial cells and on the antigen presentation of T cells. For instance, it inhibits the phagocytic activity of neutrophils and alters cytokine production of monocytes. [14]
Entry of edema toxin in the host cell
|
The edema factor has a 30 kDa protective antigen-binding domain at its
The coloration is made according to this scheme:N | C |
This domain exposes a richly negative-charged surface which easily interacts with the positively charged residues of the protective antigen. Edema factor's protective antigen-binding domain can be divided into two subdomains. (Colors:Alpha Helices and Beta Strands )
is composed of three layers, α/β sandwich domain (four β-sheets β1 to β4, in sandwich between four α-helices α1 to α4). The domain is composed of five helices. The protective antigen-binding domain contains five joining loops L1 to L5, and L5 has the key exposed residues that bind to the protective antigen. Residues in α6, α7 and in the joining loop between α7 and α8 at the C-terminal domain are also implied in the interaction. [15]
The edema factor is delivered into host cells thanks to the protective antigen. Indeed, the protective antigen binds to cellular receptors (CMP2, capillary morphogenesis protein 2 or TEM8, tumor endothelial marker 8) and is cleaved at the sequence arginine-lysine-lysine-arginine by cell surface proteases. This proteolytic activation leads to the oligomerisation of a protective antigen heptamer. The heptamer is composed of the 63 kDa fragment. One heptamer can bind three molecules of edema factor (or lethal factor). Such a complex gets into the cell by endocytosis and finally the protective antigen helps the translocation of the edema factor from late endosome into the cytoplasm. Once it is in the host cell, the edema factor becomes membrane-associated. It is not known whether it is due to its association with calmodulin or to its binding with other cellular elements. [16]
Activation of the adenylyl cyclase activity by calmodulin
Domain organisation of the edema factor and of calmodulin
The edema factor has three domains: a protective antigen-binding domain (30 kDa at the N-terminus as seen before), a helical domain (17 kDa) and a catalytic core domain (43 kDa in the C-terminal 510 amino acid region). The catalytic core domain is itself composed of two domains called CA and CB. The helical domain and the catalytic core are linked by switch C. The adenylate cyclase catalytic site is located at the interface of CA and CB. In the absence of calmodulin the enzyme remains inactive thanks to a disordered catalytic loop at the interaction site between the helical domain and the catalytic core domain. [17]
Calmodulin has two globular domains, the N-terminal and C-terminal domains, that are connected by a flexible α-helix. Each one of these domains can bind two calcium ions thanks to two helix-loop-helix motifs. The binding of a calcium ion induces a conformational change: the domain goes from a hydrophilic "closed" conformation to an "open" state which exposes a hydrophobic pocket. This hydrophobic pocket plays an important role in the interaction of calmodulin with other molecules. [18]
Molecular basis for the activation of edema factor by calmodulin
|
the activation of anthrax adenylyl cyclase by calmodulin. (PDB 1k8t)[19]
Legend: Protein Ligand Solvent
The helical domain of the edema factor interacts with the adenylate cyclase domain and switch C in the absence of calmodulin. This locks the enzyme in an inactive state. The binding of calmodulin on four discrete regions dicrupts most of those interaction allowing the enzyme to switch in an active form. This takes place in two steps. [20]
The calcium-free, closed N-terminal domain of calmodulin binds to the edema factor thanks to an interaction with its helical domain. This interaction is due to hydrogen bonds and a salt bridge between helices I and II of the N-terminal domain of calmodulin and helices L and M of the helical domain of the edema factor. When the N-terminal domain is noud to the helical domain, the calcium-loaded C-terminal domain in its open conformation inserts between the helical domain and the catalytic core. This allows a conformational change of switch C that will stabilize the catalytic loop (switch B) of the enzyme in an active state. A rigid-body rotation of CB relative to CA also occurs. This changes the pocket formed between these two domains and allows the interaction of the edema factor with the phospates of ATP. More precisely, basic lysines of the active site (K346, K353 and K372) are moved upwards. Indeed, the edema factor helical domain undergoes a 15 Å translation and a 30° rotation away from the catalytic core. [21] [22]
Two magnesium ions are coordinated by the catalytic site
|
Adenylyl cyclase activity of the edema factor requires two magnesium ions. One of them is coordinated by The other one is coordinated by the non-esterified oxygens of α, β and γ phosphates of ATP and by aspartic D493. The distance between these two magnesium ions is about 4.5 Å. One ion deprotonates the 3'OH of ATP whereas the other one stabilizes the penta-covalent intermediate during the transition state. [23]
also plays a key role in the adenylyl cyclase reaction. Histidine is a crucial amino acid because its pKa (6.8) is close to pH of cytoplasm. It may intervene in proton-transfer reactions. Histidine 351 has a critical role in the catalytic activity of edema factor but is not a catalytic base. Since it is about 6.0 Å away from the 3'O of cAMP, its role is to allow a water molecule to enter between them. Histidine does not act as a general base (acceptor of 3'OH proton), but is facilitates an increase in the concentration of HO- ions in the proximity of the 3'OH group. [24]
Mechanism of adenylyl cyclase of edema factor
|
The current model for the catalytic reaction of edema factor is the following: the reaction is mediated by the nucleophilic attack of the 3' oxygen atom on the α-phosphate. Indeed, this oxygen in near the α-phosphate. The distance between the nucleophile 3'O and the α-phosphate atom os ATP is shorter than 3.5 Å. A 3'-endo conformation of the ribose and a direct coordination of the 3'O atom by the catalytic magnesium ion is the ideal geometry for the initiation of the nucleophilic attack of the 3'O on the α-phosphate. [25]
The adenylyl cyclase reaction takes place in several steps: binding of ATP to the edema factor, enabling the deprotonation of 3'OH, stabilization of the penta-coordinated phosphorus intermediate and finally the release of cAMP and pyrophosphate. The enzyme binds its substrate, ATP and asparagine 583 interacts with it to restrict the rotation of the ribose. 3'OH is so hold in place for its nucleophilic attack during the catalysis. Moreover, the protonated stabilizes the HO- ion near the 3'OH group. Another model is that a neutral histidine deprotonates a water molecule and that the resulting HO- ion facilitates the deprotonation of 3'OH of ATP. [26]
The magnesium ion that is coordinated by is localized near the 3'OH of ATP. Since it is positively charged, it stabilizes the negative charge of the 3'-oxyanion and thus facilitates the deprotonation of 3'OH. The action of this ion and of can be additive. To summarise, increases the local pH by attracting HO-, and the metal ion decreases the pKa of the 3'OH group. Maybe the magnesium ion also stabilizes the reaction intermediate by moving towards the non-bridging oxygen of α-phosphate durung the nucleophilic attack. [27]
The second magnesium ion, that is coordinated with the oxygens of the phosphates of ATP, facilitates the bond breakage between α and β-phosphates. Indeed, it stabilizes the resulting negative charges. The stabilisation of the negative charged intermediate is also performed by different positive charged residues: [28]
The products, pysophosphate and cyclic AMP dissociate through different solvent accessible channels in a two-step process facilitated by product protonation. They are linked to the enzyme by electrostatic interactions with the magnesium ions. The binding of a water molecule to the ions is competitive with thier binding to reaction products and breaks the electrostatic links. Ligands are progressively solvated. In a first time the most important electrostatic interactions are preserved. This depends on the flexibility of the active site. Then the electrostatic interactions are broken and the products can diffuse into solvent. [29]
Prevention and treatment
It is possible to use classical therapeutic approaches to fight anthrax disease. Since the original vaccine trials by Louis Pasteur in 1881, an attenuated Stern strain is still successfully used as a vaccine in livestock. Many human vaccines based on PA have been developed in the 1960s.
Antibodies
A large number of treatments against anthrax toxin currently in development are antibodies. The majority of these antibodies target the receptor binding domain of PA, blocking binding of PA to cellular receptors. [30]
Efforts to make antibodies to EF have had varied levels of success. Immunoglobulin G (IgG) have been produced, antibodies of moderate affinity to EF, one of which (9F5) was able to inhibit binding of EF to PA and prevent physiological effects of EF on Chinese hamster ovary (CHO) cells. [31]
Winterroth and colleagues described six antibodies of moderate affinity, including one IgM that could neutralize EF activity in CHO cells. This antibody had no significant protective effect against a Sterne strain infection in a mouse model but extended the mean time to death when combined with a subprotective dose of anti-PA antibody. [32]
Chen and colleagues developed chimpanzee antibodies that included one having very high affinity and that competes with calmodulin for binding to the helical domain of EF. This antibody was very effective at preventing ET-mediated edema in a mouse footpad model and provided significant protection in a systemic toxin challenge. [33]
Leysath et al. developed and characterized four anti-EF monoclonal antibodies (MAb). MAb bind to epitopes on three different domains of EF: PA binding domain, catalytic CB domain, and helical domain. They showed that three of the four IgGs have neutralizing activity in vitro and in vivo, inhibiting production of cAMP. Antibody 7F10 has the highest efficacy and 3F2 is the first monoclonal antibody to EF whose epitope is on the catalytic CB domain. MAb are highly specific for EF and do not cross-react with LF or PA and can decrease edema levels and progression of disease in mouse models. These antibodies can serve as reagents in diagnostics assays, and can be useful reagents for further molecular studies of EF action. [34]
Non-nucleotide inhibitors
Schein et al. identified non-nucleotide inhibitors of EF. Inhibitors targeting sites for allosteric activators have recently been identified. [35] They chose to do a direct design based on analysis of the structure of the substrate binding site of the EF protein, rather than a design starting from modifying nucleotides related to the substrate itself. Comparison of the active site conformation in various crystal structures in the Protein database (PDB) revealed how the active site of the toxin differed from the mammalian adenyl cyclase enzymes. The docking of 3'-dATP to the crystal structure of EF (PDB structure: 1K90 [36] had low RMSD (root-mean-square deviations) between the predicted structure and the crystal structure, and thus, AutoDock 3.0 was reliable enough to predict the binding mode of the ligands to EF.
Although early treatment with antibiotics can greatly reduce the effects of bacterial infections, inhibitors of the toxins could play a therapeutic role in later stage infections, in preventing toxin induced diarrhea or even death. The fluorenone based inhibitors can be useful, but it could be better to combine them with LF inhibitors to treat late stage anthrax infections, where antibiotics might be unable to prevent death. [37]
Structural comparison of AC families and the development of selective EF inhibitor
There are at least six classes of adenylyl cyclase (the classification is based on their primary sequence). Five classes are found only in bacteria and the last one, class III, exists as well in prokaryotes as in eukaryotes. Class II adenylyl cyclase are secreted by pathogenic bacteria and the edema factor belongs to this class. The catalytic site of these several classes are different. This enables the fact that some molecules could inhibit the adenylyl cyclase toxins without inhibiting those of the class III. Inhibitors can be designed to interfere either with the binding of calmodulin or with the binding of the substrate. [38]
These inhibitors could be further developed as an anti-anthrax treatment, which will be administered with antibiotics. Among those, the most potent EF inhibitor is an approved drug, Adefovir. Adefovir can selectively inhibit the activity of EF with no inhibition of the activity of endogenous host AC. Adefovir is an acyclic nucleoside and it can treat chronic hepatitis B virus infection. Tenofovir, another acyclic nucleoside which is a drug against human immunodeficiency virus, also show high affinity to EF. [39]
About this Structure
1lvc is a 6 chain structure with sequence from Bacillus anthracis and Homo sapiens. Full crystallographic information is available from OCA.
See Also
Reference
- ↑ Leppla SH. Anthrax toxin edema factor: a bacterial adenylate cyclase that increases cyclic AMP concentrations of eukaryotic cells. Proc Natl Acad Sci U S A. 1982 May;79(10):3162-6. PMID:6285339
- ↑ Fouet, A. 2009. The surface of Bacillus anthracis. Mol. Aspects Med. 30:374–385
- ↑ Moayeri, M., and S. H. Leppla. 2009. Cellular and systemic effects of anthrax lethal toxin and edema toxin. Mol. Aspects Med. 30:439–455
- ↑ http://www.rcsb.org/pdb/101/motm_disscussed_entry.do?id=1k90
- ↑ Fouet, A. 2009. The surface of Bacillus anthracis. Mol. Aspects Med. 30:374–385
- ↑ Moayeri, M., and S. H. Leppla. 2009. Cellular and systemic effects of anthrax lethal toxin and edema toxin. Mol. Aspects Med. 30:439–455
- ↑ Tournier, J.N.; Rossi Paccani, S.; Quesnel-Hellmann, A.; Baldari, C.T. Anthrax toxins: A weapon to systematically dismantle the host immune defenses. Mol. Aspects Med. 2009, 30, 456–466
- ↑ Klimpel, K. R., N. Arora, and S. H. Leppla. 1994. Anthrax toxin lethal factor contains a zinc metalloprotease consensus sequence which is required for lethal toxin activity. Mol. Microbiol. 13:1093–1100
- ↑ Leppla, 1982, Anthrax toxin edema factor: a bacterial adenylate cyclase that increases cAMP concentration in eukaryotic cells. Proc. Natl. Acad. Sci. USA 79:3162-3163
- ↑ http://www.ncbi.nlm.nih.gov/pmc/articles/PMC3064363/
- ↑ http://en.wikipedia.org/wiki/Anthrax_toxin
- ↑ Leppla SH. Anthrax toxin edema factor: a bacterial adenylate cyclase that increases cyclic AMP concentrations of eukaryotic cells. Proc Natl Acad Sci U S A. 1982 May;79(10):3162-6. PMID:6285339
- ↑ Leppla SH. Anthrax toxin edema factor: a bacterial adenylate cyclase that increases cyclic AMP concentrations of eukaryotic cells. Proc Natl Acad Sci U S A. 1982 May;79(10):3162-6. PMID:6285339
- ↑ Tang WJ, Guo Q. The adenylyl cyclase activity of anthrax edema factor. Mol Aspects Med. 2009 Dec;30(6):423-30. doi: 10.1016/j.mam.2009.06.001. Epub 2009, Jun 26. PMID:19560485 doi:10.1016/j.mam.2009.06.001
- ↑ Shen Y, Zhukovskaya NL, Guo Q, Florian J, Tang WJ. Calcium-independent calmodulin binding and two-metal-ion catalytic mechanism of anthrax edema factor. EMBO J. 2005 Mar 9;24(5):929-41. Epub 2005 Feb 17. PMID:15719022
- ↑ Tang WJ, Guo Q. The adenylyl cyclase activity of anthrax edema factor. Mol Aspects Med. 2009 Dec;30(6):423-30. doi: 10.1016/j.mam.2009.06.001. Epub 2009, Jun 26. PMID:19560485 doi:10.1016/j.mam.2009.06.001
- ↑ Tang WJ, Guo Q. The adenylyl cyclase activity of anthrax edema factor. Mol Aspects Med. 2009 Dec;30(6):423-30. doi: 10.1016/j.mam.2009.06.001. Epub 2009, Jun 26. PMID:19560485 doi:10.1016/j.mam.2009.06.001
- ↑ Shen Y, Zhukovskaya NL, Guo Q, Florian J, Tang WJ. Calcium-independent calmodulin binding and two-metal-ion catalytic mechanism of anthrax edema factor. EMBO J. 2005 Mar 9;24(5):929-41. Epub 2005 Feb 17. PMID:15719022
- ↑ http://www.rcsb.org/pdb/explore/explore.do?structureId=1k8t
- ↑ Tang WJ, Guo Q. The adenylyl cyclase activity of anthrax edema factor. Mol Aspects Med. 2009 Dec;30(6):423-30. doi: 10.1016/j.mam.2009.06.001. Epub 2009, Jun 26. PMID:19560485 doi:10.1016/j.mam.2009.06.001
- ↑ Tang WJ, Guo Q. The adenylyl cyclase activity of anthrax edema factor. Mol Aspects Med. 2009 Dec;30(6):423-30. doi: 10.1016/j.mam.2009.06.001. Epub 2009, Jun 26. PMID:19560485 doi:10.1016/j.mam.2009.06.001
- ↑ Shen Y, Zhukovskaya NL, Guo Q, Florian J, Tang WJ. Calcium-independent calmodulin binding and two-metal-ion catalytic mechanism of anthrax edema factor. EMBO J. 2005 Mar 9;24(5):929-41. Epub 2005 Feb 17. PMID:15719022
- ↑ Shen Y, Zhukovskaya NL, Guo Q, Florian J, Tang WJ. Calcium-independent calmodulin binding and two-metal-ion catalytic mechanism of anthrax edema factor. EMBO J. 2005 Mar 9;24(5):929-41. Epub 2005 Feb 17. PMID:15719022
- ↑ Shen Y, Zhukovskaya NL, Guo Q, Florian J, Tang WJ. Calcium-independent calmodulin binding and two-metal-ion catalytic mechanism of anthrax edema factor. EMBO J. 2005 Mar 9;24(5):929-41. Epub 2005 Feb 17. PMID:15719022
- ↑ Shen Y, Zhukovskaya NL, Guo Q, Florian J, Tang WJ. Calcium-independent calmodulin binding and two-metal-ion catalytic mechanism of anthrax edema factor. EMBO J. 2005 Mar 9;24(5):929-41. Epub 2005 Feb 17. PMID:15719022
- ↑ Shen Y, Zhukovskaya NL, Guo Q, Florian J, Tang WJ. Calcium-independent calmodulin binding and two-metal-ion catalytic mechanism of anthrax edema factor. EMBO J. 2005 Mar 9;24(5):929-41. Epub 2005 Feb 17. PMID:15719022
- ↑ Shen Y, Zhukovskaya NL, Guo Q, Florian J, Tang WJ. Calcium-independent calmodulin binding and two-metal-ion catalytic mechanism of anthrax edema factor. EMBO J. 2005 Mar 9;24(5):929-41. Epub 2005 Feb 17. PMID:15719022
- ↑ Shen Y, Zhukovskaya NL, Guo Q, Florian J, Tang WJ. Calcium-independent calmodulin binding and two-metal-ion catalytic mechanism of anthrax edema factor. EMBO J. 2005 Mar 9;24(5):929-41. Epub 2005 Feb 17. PMID:15719022
- ↑ Martinez L, Malliavin TE, Blondel A. Mechanism of reactant and product dissociation from the anthrax edema factor: a locally enhanced sampling and steered molecular dynamics study. Proteins. 2011 May;79(5):1649-61. doi: 10.1002/prot.22991. Epub 2011 Mar 21. PMID:21425348 doi:10.1002/prot.22991
- ↑ Young, J. A., and R. J. Collier. 2007. Anthrax toxin: receptor-binding, internalization, pore formation, and translocation. Annu. Rev. Biochem. 76:243–265
- ↑ Little, S. F., S. H. Leppla, J. W. Burnett, and A. M. Friedlander. 1994. Structure-function analysis of Bacillus anthracis edema factor by using monoclonal antibodies. Biochem. Biophys. Res. Commun. 199:676–682
- ↑ Winterroth, L., J. Rivera, A. S. Nakouzi, E. Dadachova, and A. Casadevall. 2010. A neutralizing monoclonal antibody to edema toxin and its effect on murine anthrax. Infect. Immun. 78:2890–2896
- ↑ Chen, Z., et al. 2009. Potent neutralization of anthrax edema toxin by a humanized monoclonal antibody that competes with calmodulin for edema factor binding. Proc. Natl. Acad. Sci. U. S. A. 106:13487–13492
- ↑ Leysath, C.E. et al., Mouse Monoclonal Antibodies to Anthrax Edema Factor Protect against Infection. Infection and immunity, Nov. 2011, p. 4609–4616
- ↑ Laine, É.; Martínez, L.; Ladant, D.; Malliavin, T.; Blondel, A. Molecular motions as a drug target: Mechanistic simulations of anthrax toxin edema factor function led to the discovery of novel allosteric inhibitors. Toxins 2012, 4, 580–604
- ↑ Drum, C.L.; Yan, S.Z.; Bard, J.; Shen, Y.Q.; Lu, D.; Soelaiman, S.; Grabarek, Z.; Bohm, A.; Tang, W.J. Structural basis for the activation of anthrax adenylyl cyclase exotoxin by calmodulin. Nature 2002, 415, 396–402
- ↑ Schein, C.H. et al., Pharmacophore Selection and Redesign of Non-nucleotide Inhibitors of Anthrax Edema Factor. Toxins 2012, 4, 1288-1300; doi:10.3390/toxins4111288
- ↑ Shen et al. 2004
- ↑ Suryanarayana et al., Distinct Interactions of 2′- and 3′-O-(N-Methyl)anthraniloyl-Isomers of ATP and GTP with the Adenylyl Cyclase Toxin of Bacillus anthracis, Edema Factor, Biochem Pharmacol. 2009 August 1; 78(3): 224–230
Proteopedia Page Contributors and Editors
Charlotte Kern - Aude Zimmermann ESBS