User:Jessica Sheehe/Sandbox 1
From Proteopedia
cGMP-dependent protein kinase I alpha (PKG-Ia)
|
I. Introduction
cGMP-dependent protein kinase (PKG) (EC2.7.11.12) is a member of the AGC kinase family that mediates intracellular signaling through serine and threonine phosphorylation. PKG family members are encoded by two genes: PKGI and PKGII. A splice variant in PKGI leads to expression of PKG-Ia and PKG-Iβ (1). Both PKGI isoforms are found in smooth muscle cells, platelets, cerebellum, and hippocampus, however the PKG-Ia isoform is preferentially located in the heart and lungs as well (2). cGMP, produced from guanylate cyclase through activation by natriuretic peptides or NO, is required for full activation of PKG-Ia (1).
II. Isoforms and Homology
There are two genes that code for PKG—PRKG1 and PRKG2. Alternative splicing of the PRKG1 results in PKG-Ia and PKG-Ib isoforms that differ at their N-terminal region by approximately 65%(1, 3). ClustalW sequence alignment
III. General Structure
PKG-Ia is a dimer. Each monomer consists of a dimerization domain, an autoinhibitory domain, two cyclic nucleotide binding sites (A site and B site), a switch helical domain, and a catalytic domain (4).
IV. Dimerization Domain
Dimerization of PKG-Ia is mediated through its leucine zipper motif located at residues 9-44 in the N-terminus of the enzyme (5). The zipper also mediates targeting to substrates leading to their phosphorylation (2). Leucine zippers are right-handed helices that interact through their leucine and isoleucine residues forming a left-handed coiled coil. The primary structure is characterized by a heptad repeat of amino acids (abcdefg) where residues a and d are typically hydrophobic and residues e and g are typically hydrophilic. The structure of the PKG-Ia leucine zipper was solved by NMR in 2005 by Schnell and colleagues (PDB 1ZXA). Using COILS and PARCOILS they predicted the parallel structure of the zipper (6). The helical wheel diagram created using DrawCoil1.0 indicates the residues at the dimer interface. In the corresponding sequence, residues in the a and d positions of the heptad repeat are colored green and the e and g positions are colored purple, indicating the predominant residues at the interface are hydrophobic leucines and isoleucines however the structure is also stabilized by hydrophilic residues in the e and g positions that associate through hydrogen bonding and shield the hydrophobic core.
V. Autoinhibitory Site
PKG-Ia contains a autoinhibitory site that spans residues 50-67 and is denoted by the sequence STHIGPRTTRAQGISAEP (7). The site was identified due to its homology to the autoinhibitory site of PKA (8). The residues RAQGISAEP are thought to act as a pseudosubstrate by binding to the catalytic domain in the substrate binding groove to inhibit enzyme activity (9). Binding of the pseudosubstrate is primarily mediated by autophosphorylation (10). Aitken and colleagues identified three autophosphorylation sites on PKG-Ia after incubation with cAMP: Ser50, Thr58, and Ser64 (11). In a study by Busch and colleagues, Ser64 is a conserved residue that is important for autoinhibition and lowering PKG-Ia binding affinity to cGMP, regardless. Mutation to Ala and Thr did not change activity while mutation to Asp or Asn resulted in an almost constitutively active enzyme. These data suggest that autophosphorylation at Ser64 results in stearic hindrance that blocks binding of the pseudosubstrate to the catalytic site and is not due to electrostatic interaction (3). The hydrophobicity of Ile63 was also found to be important for maintaining the inactive state of PKG-Ia. According to Yuasa and colleagues, mutation to Phe, Val, or Leu did not result in a significant decrease in inhibition while mutation to hydrophilic residues such as Gly, Ala, Thr, and Ser abolished inhibitory effects (8).
VI. Regulatory Domain and Switch Helical Subdomain
The is composed of two cyclic nucleotide binding sites per monomer of PKG-Ia. The A site includes residues 87-210 binds cAMP or cGMP and is considered the fast binding site. The B site includes residues 211-328 and specificalluy binds cGMP, however is considered the slowing binding site (4, 10). Binding of two cGMP molecules to the regulatory domain leads to activation of the kinase.
The structure of the regulatory domain was determined by X-ray crystallography at 2.5Å resolution (PDB 3SHR). to the A site and confirmed the important residues for cyclic nucleotide binding. One oxygen of the phosphate group binds to the backbone amide of Ala169 and to the side chain of Arg176 through electrostatic interactions of 3.2Å and 3.0Å respectively. The second oxygen of the phosphate group of cAMP binds to the backbone amide and side chain of Thr177 with bond distances of 2.7Å and 2.5Å respectively. Additionally, the hydroxyl group of cAMP attached to the sugar hydrogen bonds with the backbone amide of Gly166. These interactions stabilize the binding of cyclic nucleotide to the A site of PKG-Ia (10).
Another interesting feature of PKG-Ia is the switch helical subdomain spanning residues 328-355 (PDB 3SHR). In the , the hydrophobic tip of the switch helix in the first protomer binds to a hydrophobic knob of the opposing protomer forming a site of interchain communication that is thought to facilitate crosstalk between the regulatory domains.
VII. Catalytic Site
The exact 3D configuration of the catalytic domain of PKG-Ia is unknown, however it is thought to have a similar shape to PKA. AGC kinases have conserved tertiary structure in their catalytic domains, suggesting that PKG-Ia, as a member of the AGC kinase family, would also conform to a similar 3D structure (12). The catalytic domains of PKG-Ia and PKA have 45% sequence identity according to NCBI’s Protein Blast. In PKA, the N and C lobes sandwich the ATP and substrate for catalysis to occur. Conserved structural features include the glycine rich loop, the catalytic loop, and the activation loop. In PKA, the glycine rich loop contains the sequence motif GxGGxG and forms a pocket for ATP to bind. The catalytic loop motif denoted by the sequence YRDLKxxN is involved in coordinating the phosphotransferase reaction. The activation loop starts with DFG motif and ends with an APE motif (13). The APE sequence is highly conserved among protein kinases (14). Phosphorylation of the activation loop is required for kinase activity. Phosphorylation has been documented on Thr517; mutation of this residue to Ala results in a catalytically dead kinase (15).
VIII. Phosphorylation of PKG-Ia
According to phosphosite.org, there are eleven previously documented phosphorylations on PKG-Ia: one in the dimerization domain, six in the autoinhibitory site, one in the B site of the regulatory domain, and three in the catalytic domain located on the activation loop. Many of these were discovered by proteomic discovery mass spectrometry only and have not been further characterized. S45 (16), S51 (16, 17), S59 (16, 18, 19), S65 (3, 16, 17, 20), T85 (16, 17), and T517 (15, 21) were discovered by site specific mutagenesis.
IX. Evolutionary Conservation
The sequence of PKG-Ia is highly conserved among species. Sequence alignment of 12 species (human, bovine, mouse, rat, wild boar, Japanese rice fish, Zebra fish, fruit fly, honey bee, and silk worm) using ClustalW indicated high sequence homology throughout. About 75% of the residues showed invariant or homologous residues. There were 341 invariant residues found in all species, which is about 50% of the entire human PKG-Ia sequence. The region with the most variability was the N-terminus, which interesting has highest region of variability among PKG isoforms.
X. PDB Structures
PDB Code | Residues | Method | Resolution (Å) | Ligands | Citation |
---|---|---|---|---|---|
3SHR | 78-355 | X-ray Diffraction | 2.50 | cAMP
SO4 | Structure (2011) 19:1317-1327 |
3OD0 | 92-227 | X-ray Diffraction | 2.90 | cGMP | Plos One (2011) 6: e18413-e18413 |
3OCP | 92-227 | X-ray Diffraction | 2.49 | cAMP | Plos One (2011) 6: e18413-e18413 |
3OGJ | 92-227 | X-ray Diffraction | 2.75 | cAMP
PO4 | Plos One. (2011) 6: e18413-e18413 |
4KU7 | 204-354 | X-ray Diffraction | 1.65 | Iodide
cGMP | Structure. (2014) 22: 116-124 |
1ZXA | 1-58 | NMR | ——— | ——— | Protein Sci. (2005) 14: 2421-2428 |
XI. Other Interesting Structural Features
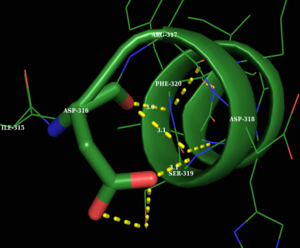
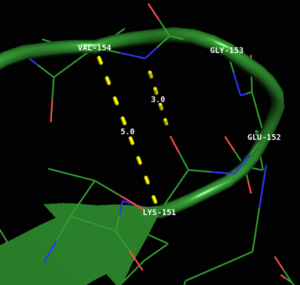
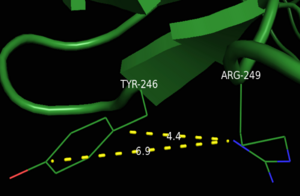
References
1. Hofmann F, Bernhard D, Lukowski R, Weinmeister P (2009) cGMP regulated protein kinases (cGK). Handb Exp Pharmacol:137–62. A
2. Hofmann F (2005) The biology of cyclic GMP-dependent protein kinases. J Biol Chem 280:1–4.
3. Busch JL, Bessay EP, Francis SH, Corbin JD (2002) A conserved serine juxtaposed to the pseudosubstrate site of type I cGMP-dependent protein kinase contributes strongly to autoinhibition and lower cGMP affinity. J Biol Chem 277:34048–54.
4. Moon TM, Osborne BW, Dostmann WR (2013) The switch helix: a putative combinatorial relay for interprotomer communication in cGMP-dependent protein kinase. Biochim Biophys Acta 1834:1346–51.
5. Kato M et al. (2012) Direct binding and regulation of RhoA protein by cyclic GMP-dependent protein kinase Iα. J Biol Chem 287:41342–51.
6. Schnell JR, Zhou G-P, Zweckstetter M, Rigby AC, Chou JJ (2005) Rapid and accurate structure determination of coiled-coil domains using NMR dipolar couplings: application to cGMP-dependent protein kinase Ialpha. Protein Sci 14:2421–8. 7. Ruth P et al. (1997) Identification of the amino acid sequences responsible for high affinity activation of cGMP kinase Ialpha. J Biol Chem 272:10522–8.
8. Yuasa K, Michibata H, Omori K, Yanaka N (2000) Identification of a conserved residue responsible for the autoinhibition of cGMP-dependent protein kinase Ialpha and beta. FEBS Lett 466:175–8.
9. Kemp BE, Parker MW, Hu S, Tiganis T, House C (1994) Substrate and pseudosubstrate interactions with protein kinases: determinants of specificity. Trends Biochem Sci 19:440–444.
10. Osborne BW et al. (2011) Crystal structure of cGMP-dependent protein kinase reveals novel site of interchain communication. Structure 19:1317–27.
11. Aitken A, Hemmings BA, Hofmann F (1984) Identification of the residues on cyclic GMP-dependent protein kinase that are autophosphorylated in the presence of cyclic AMP and cyclic GMP. Biochim Biophys Acta 790:219–25.
12. Hofmann F, Dostmann W, Keilbach A, Landgraf W, Ruth P (1992) Structure and physiological role of cGMP-dependent protein kinase. Biochim Biophys Acta 1135:51–60.
13. Steichen JM et al. (2012) Structural basis for the regulation of protein kinase A by activation loop phosphorylation. J Biol Chem 287:14672–80.
14. Eldar-Finkelman H, Eisenstein M (2009) Peptide inhibitors targeting protein kinases. Curr Pharm Des 15:2463–70.
15. Feil R, Kellermann J, Hofmann F (1995) Functional cGMP-dependent protein kinase is phosphorylated in its catalytic domain at threonine-516. Biochemistry 34:13152–8.
16. Pinkse MWH, Uitto PM, Hilhorst MJ, Ooms B, Heck AJR (2004) Selective isolation at the femtomole level of phosphopeptides from proteolytic digests using 2D-NanoLC-ESI-MS/MS and titanium oxide precolumns. Anal Chem 76:3935–43.
17. Dey NB, Busch JL, Francis SH, Corbin JD, Lincoln TM (2009) Cyclic GMP specifically suppresses Type-Ialpha cGMP-dependent protein kinase expression by ubiquitination. Cell Signal 21:859–66. 18. Hou Y, Lascola J, Dulin NO, Ye RD, Browning DD (2003) Activation of cGMP-dependent protein kinase by protein kinase C. J Biol Chem 278:16706–12.
19. Takio K, Smith SB, Walsh KA, Krebs EG, Titani K (1983) Amino acid sequence around a “hinge” region and its “autophosphorylation” site in bovine Lung cGMP-dependent protein kinase. J Biol Chem 258:5531–6.
20. Smith JA, Reed RB, Francis SH, Grimes K, Corbin JD (2000) Distinguishing the roles of the two different cGMP-binding sites for modulating phosphorylation of exogenous substrate (heterophosphorylation) and autophosphorylation of cGMP-dependent protein kinase. J Biol Chem 275:154–8.
21. Browning DD, McShane MP, Marty C, Ye RD (2000) Nitric oxide activation of p38 mitogen-activated protein kinase in 293T fibroblasts requires cGMP-dependent protein kinase. J Biol Chem 275:2811–6.